About
What We Do
• Custom manufacturing that meets your company specifications.
• Quality pricing.
• Just-in time delivery.
• Quality assurance: selected (PAC) materials meet AWWA NSF Standards.
• Experience: Over 20 years in activated carbon market.
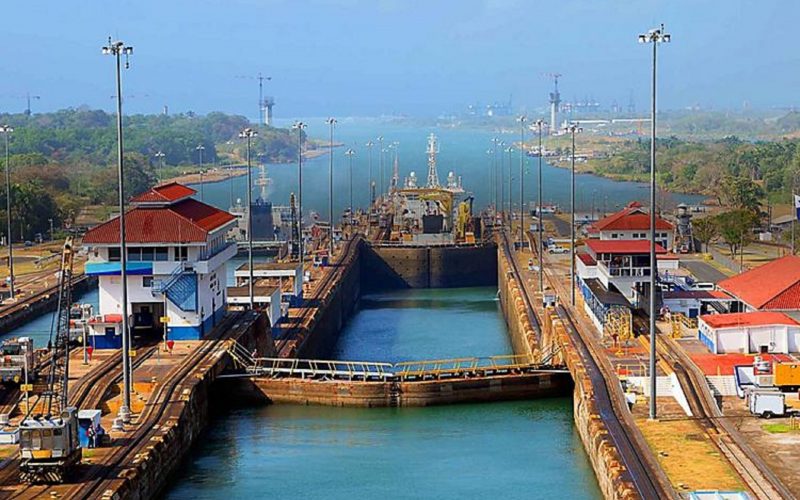
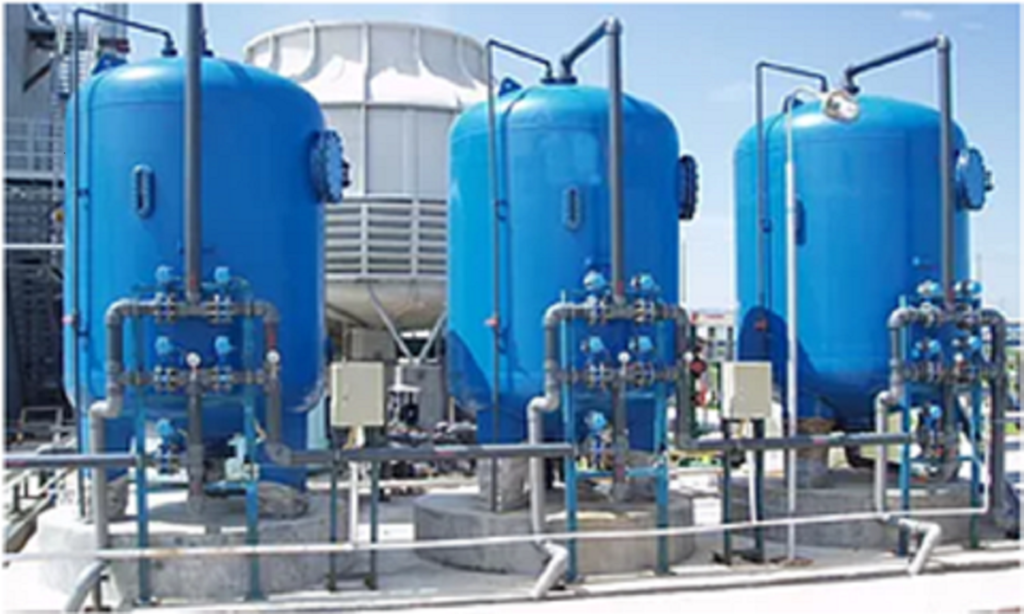
Personalized Solutions
Our primary advantage is low competitive prices.
Our technical staff can custom-match your Activated Carbon specifications.
With our just-in-time delivery, we ship directly to your location from our primary manufacturing sites in China, South America and USA warehouses, making our prices very competitive.
Our Services
Are primarily geared towards large industrial, municipal, governmental applications and water treatment equipment manufactures and chemical plants. Our Custom Activated Carbon “MATCHING SYSTEM“, makes us unique and sets us apart from our competition. This involves the design of Activated Carbon that will meet your requirements and system application.
What Is Activated Carbon?
Activated carbon has an incredibly large surface area per unit volume, and a network of submicroscopic pores where adsorption takes place. Activated carbon is a material that is produced from carbonaceous source materials, such as coal, coconuts, nutshells, peat, wood, and lignite. The primary raw material used for activated carbon is any organic material with a high carbon content. The carbon-based material is converted to activated carbon through physical modification and thermal decomposition in a furnace, under a controlled atmosphere and temperature. The finished product has a large surface area per unit volume and a network of submicroscopic pores where adsorption takes place.
“Activated Carbon“, also called activated charcoal or activated coal is a form of carbon that has been processed to make it extremely porous and thus to have a very large surface area available for adsorption or chemical reactions.
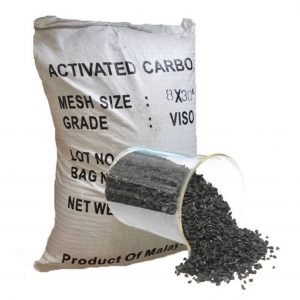
Show More
James Dewar, the scientist after which the Dewar (Vacuum flask) is named, spent much time studying activated carbon and published a paper regarding its absorption capacity with regard to gases. In this paper, he discovered that cooling the carbon to liquid nitrogen temperatures allowed it to absorb significant quantities of numerous air gases, among others, that could then be recollected by simply allowing the carbon to warm again and that coconut based carbon was superior for the effect. He uses oxygen as an example, wherein the activated carbon would typically absorb the atmospheric concentration (21%) under standard conditions, but release over 80% oxygen if the carbon was first cooled to low temperatures.
Physically, activated carbon binds materials by van der Waals force or London dispersion force.
Activated carbon does not bind well to certain chemicals, including alcohols, glycols, strong acids and bases, metals and most inorganics, such as lithium, sodium, iron, lead, arsenic, fluorine, and boric acid.
Activated carbon does adsorb iodine very well and in fact the iodine number, mg/g, (ASTM D28 Standard Method test) is used as an indication of total surface area.
Contrary to a claim throughout the web, activated carbon can capture gaseous ammonia. In fact, it is utilized for that very function in many diazo copier machines.
Carbon monoxide is not well absorbed by activated carbon. This should be of particular concern to those using the material in filters for respirators, fume hoods or other gas control systems as the gas is undetectable to the human senses, toxic to metabolism and neurotoxic.
Substantial lists of the common industrial and agricultural gases absorbed by activated carbon can be found online.
Activated carbon can be used as a substrate for the application of various chemicals to improve the adsorptive capacity for some inorganic (and problematic organic) compounds such as hydrogen sulfide (H2S), ammonia (NH3), formaldehyde (HCOH), radioisotopes iodine-131(131I) and mercury (Hg). This property is known as chemisorption.

Coconut Shell Based
Coconut Shell based Activated Carbon products are well known for their signature physical properties.
Very large internal surface areas characterized by micro porosity along with relatively high hardness and low dust make these carbons particularly attractive for water and critical air applications such as point of use water filters and respirators respectively.
Carbon Solutions, LLC., as a manufacturer and distributor of Coconut Shell based carbons stocks a wide range of high quality products. In addition to granular forms, we hold a number of pelletized grades (3mm, 4mm & 5mm) available for delivery to any destination worldwide.
◾ Very high surface area characterized by a large proportion of micro pores
◾ High hardness with low dust generation.
◾ Excellent purity, with most products exhibiting no more than 3-5% ash content.
◾ Renewable and green raw material.
◾ Material can be acid washed down to 1% ash content.

Coal Based
Coal based Activated Carbon products are commonly used across a large number of industries for a variety of applications.
Demand is typically high for this relatively low cost filter media in both gas and liquid applications.
Carbon Solutions, LLC. stocks a large variety of common granular and pelletized grades (3mm, 4mm & 5mm), in addition to powder carbon. We have excellent sourcing capabilities for a number of specialist coal based activated carbons available for direct shipment in container loads to worldwide customer destinations
High surface area characterized by a proportion of both mesopore (A mesoporous material is a material containing pores with diameters between 2 and 50 nm. Porous materials are classified into several kinds by their size) and micropores (A microporous material is a material containing pores with diameters less than 2 nm).
◾Consistent density
◾Hard materials with minimal dust generation.
◾Economical

Wood Based
Wood based Activated Carbons are a growing product finding use in a number of new applications in addition to producing different performance characteristics in industrial applications typically catered for by coal or coconut products.
Wood carbons have excellent decolorizing properties owing to their signature porosimetry.
High surface area characterized by a large proportion of mesopores and macropores
◾Relatively low density
◾Renewable source of raw material
In-house expertise is available for selection of the optimum carbon for any application.

Anthracite Based
Anthracite filter media is a hard, compact variety of coal that has a high luster. It has the highest carbon content, the fewest impurities, and the highest calorific content of all types of coal, which also include bituminous coal and lignite.
Anthracite promotes higher service flow rates and longer filter runs with less head loss than single media filter beds. Backwash rates are reduced as well. The filter medium is usually a 15-30 in. deep bed of sand or anthracite particle bed supports the filter media to prevent fine sand or anthracite from escaping into the underdrain system. The support bed also serves to distribute backwash water. Typical support beds consist of 1 8-1 in. gravel or anthracite in graded layers to a depth of 12-16 in.
More on Activated Carbon
Activated carbon, also called activated charcoal or activated coal, is a form of carbon that has been processed to have small, low-volume pores that make it extremely porous and thus to have a very large surface area available that increase the surface area available for adsorption or chemical reactions.
The word Activated is sometimes substituted with active.
Activated carbon is usually derived from charcoal. When derived from coal it is referred to as activated coal.
Due to its high degree of microporosity, one gram of activated carbon has a surface area in excess of 3,000 m2 (32,000 sq ft) as determined by gas adsorption. An activation level sufficient for useful applications may be obtained solely from high surface area. Further chemical treatment often enhances adsorption properties.
Carbon aerogels, while more expensive, have even higher surface areas, and are used in special applications.
Under an electron microscope, the high surface-area structures of activated carbon are revealed. Individual particles are intensely convoluted and display various kinds of porosity; there may be many areas where flat surfaces of graphite-like material run parallel to each other, separated by only a few nanometers or so. These micro pores provide superb conditions for adsorption to occur, since adsorbing material can interact with many surfaces simultaneously. Tests of adsorption behavior are usually done with nitrogen gas at 77 K under high vacuum, but in everyday terms activated carbon is perfectly capable of producing the equivalent, by adsorption from its environment, liquid water from steam at 100 °C and a pressure of 1/10,000 of an atmosphere.
Many carbons preferentially adsorb small molecules. Iodine number is the most fundamental parameter used to characterize activated carbon performance. It is a measure of activity level (higher number indicates higher degree of activation), often reported in mg/g (typical range 500–1200 mg/g). It is a measure of the micro pore content of the activated carbon (0 to 20 Å, or up to 2 nm) by adsorption of iodine from solution. It is equivalent to surface area of carbon between 900 m²/g and 1100 m²/g. It is the standard measure for liquid phase applications.
Iodine number is defined as the milligrams of iodine adsorbed by one gram of carbon when the iodine concentration in the residual filtrate is 0.02 normal. Basically, iodine number is a measure of the iodine adsorbed in the pores and, as such, is an indication of the pore volume available in the activated carbon of interest. Typically, water treatment carbons have iodine numbers ranging from 600 to 1100. Frequently, this parameter is used to determine the degree of exhaustion of a carbon in use. However, this practice should be viewed with caution as chemical interactions with the adsorbate may affect the iodine uptake giving false results. Thus, the use of iodine number as a measure of the degree of exhaustion of a carbon bed can only be recommended if it has been shown to be free of chemical interactions with adsorbates and if an experimental correlation between iodine number and the degree of exhaustion has been determined for the particular application.
Some carbons are more adept at adsorbing large molecules. Molasses number or molasses efficiency is a measure of the mesopore content of the activated carbon (greater than 20 Å, or larger than 2 nm) by adsorption of molasses from solution. A high molasses number indicates a high adsorption of big molecules (range 95–600). Caramel dp (decolorizing performance) is similar to molasses number. Molasses efficiency is reported as a percentage (range 40%–185%) and parallels molasses number (600 = 185%, 425 = 85%). The European molasses number (range 525–110) is inversely related to the North American molasses number.
Molasses Number is a measure of the degree of decolorization of a standard molasses solution that has been diluted and standardized against standardized activated carbon. Due to the size of color bodies, the molasses number represents the potential pore volume available for larger adsorbing species. As all of the pore volume may not be available for adsorption in a particular waste water application, and as some of the adsorbate may enter smaller pores, it is not a good measure of the worth of a particular activated carbon for a specific application. Frequently, this parameter is useful in evaluating a series of active carbons for their rates of adsorption. Given two active carbons with similar pore volumes for adsorption, the one having the higher molasses number will usually have larger feeder pores resulting in more efficient transfer of adsorbate into the adsorption space.
Tannins are a mixture of large and medium size molecules. Carbons with a combination of macropores and mesopores adsorb tannins. The ability of a carbon to adsorb tannins is reported in parts per million concentration (range 200 ppm–362 ppm).
Some carbons have a mesopore (20 Å to 50 Å, or 2 to 5 nm) structure which adsorbs medium size molecules, such as the dye methylene blue. Methylene blue adsorption is reported in g/100g (range 11–28 g/100g).
Some carbons are evaluated based on the dechlorination half-value length, which measures the chlorine-removal efficiency of activated carbon. The dechlorination half-value length is the depth of carbon required to reduce the chlorine level of a flowing stream from 5 ppm to 3.5 ppm. A lower half-value length indicates superior performance.
The solid or skeletal density of activated carbons will typically range between 2000 and 2100 kg/m3 (125–130 lbs./cubic foot). However, a large part of an activated carbon sample will consist of air space between particles, and the actual or apparent density will therefore be lower, typically 400 to 500 kg/m3 (25–31 lbs./cubic foot).
Higher density provides greater volume activity and normally indicates better-quality activated carbon. ASTM D 2854-09 (2014) is used to determine the apparent density of activated carbon.
It is a measure of the activated carbon’s resistance to attrition. It is important indicator of activated carbon to maintain its physical integrity and withstand frictional forces imposed by backwashing, etc. There are large differences in the hardness of activated carbons, depending on the raw material and activity level.
It reduces the overall activity of activated carbon. It reduces the efficiency of reactivation. The metal oxides (Fe2O3) can leach out of activated carbon resulting in discoloration. Acid/water soluble ash content is more significant than total ash content. Soluble ash content can be very important for aquarists, as ferric oxide can promote algal growths, a carbon with a low soluble ash content should be used for marine, freshwater fish and reef tanks to avoid heavy metal poisoning and excess plant/algal growth. ASTM D2866 (Standard Method test) is used to determine the ash content of activated carbon.
Measurement of the porosity of an activated carbon by the adsorption of saturated carbon tetrachloride vapor.
The finer the particle sizes of an activated carbon, the better the access to the surface area and the faster the rate of adsorption kinetics. In vapor phase systems this needs to be considered against pressure drop, which will affect energy cost. Careful consideration of particle size distribution can provide significant operating benefits. However, in the case of using activated carbon for adsorption of minerals such as gold, the particle size should be in the range of 3.35–1.4 millimetres (0.132–0.055 in). Activated carbon with particle size less than 1 mm would not be suitable for elution (the stripping of mineral from an activated carbon).
Activated carbon is used in gas purification, gold purification, metal extraction, water purification, medicine, sewage treatment, air filters in gas masks and respirators, filters in compressed air and many other applications.
One major industrial application involves use of activated carbon in the metal finishing field. It is very widely employed for purification of electroplating solutions. For example, it is a main purification technique for removing organic impurities from bright nickel plating solutions. A variety of organic chemicals are added to plating solutions for improving their deposit qualities and for enhancing properties like brightness, smoothness, ductility, etc. Due to passage of direct current and electrolytic reactions of anodic oxidation and cathodic reduction, organic additives generate unwanted break down products in solution. Their excessive build up can adversely affect the plating quality and physical properties of deposited metal. Activated carbon treatment removes such impurities and restores plating performance to the desired level.
Activated carbon is used either as a coating on seeds or mixed with fertilizers (as in ryegrass production) to protect the seed from harmful herbicides and fungicides and improve crop yield. ... In many cases, our products can be mixed with soil to leach out excess herbicides or fungicides in the field due to spillage.
Activated Carbon for removal of toxic compounds in liquid, gas and soil applications. Its state-of-the-art manufacturing facility, located in Northern Florida, utilizes a reliable supply of reclaimed wood in combination with a proprietary activation process to generate high performance Activated Carbon products.
Powdered activated carbon is used widely as a Soil Amendment in agricultural applications. Where over-spraying of herbicides and pesticides or over-application of fertilizer contaminates the soil, Activated Carbon readily adsorbs excess organic toxic compounds before re-seeding.
Activated Carbon is commonly used to decontaminate the soil making it a cost effective choice when replanting turfgrass and food crops.
Activated carbon, in 50% w/w combination with celite, is used as stationary phase in low-pressure chromatographic separation of carbohydrates (mono-, di- trisacchardes) using ethanol solutions (5–50%) as mobile phase in analytical or preparative protocols.
Activated carbon is commonly used to purify homemade non-dangerous chemicals such as sodium acetate.
See also: Lincoln County Process
Activated carbon filters can be used to filter vodka and whiskey of organic impurities which can affect color, taste, and odor. Passing organically impure vodka through an activated carbon filter at the proper flow rate will result in vodka with an identical alcohol content and significantly increased organic purity, as judged by odor and taste.
Activated carbon is usually used in water filtration systems.
Carbon adsorption has numerous applications in removing pollutants from air or water streams both in the field and in industrial processes such as:
•Spill cleanup
•Groundwater remediation
•Drinking water filtration
•Air purification
•Volatile organic compounds capture from painting, dry cleaning, gasoline dispensing operations, and other processes.
Activated carbon is also used for the measurement of radon concentration in air.
Research is being done testing various activated carbons' ability to store natural gas and hydrogen gas. The porous material acts like a sponge for different types of gasses. The gas is attracted to the carbon material via Van der Waals forces. Some carbons have been able to achieve bonding energies of 5–10 kJ per mol. The gas may then be desorbed when subjected to higher temperatures and either combusted to do work or in the case of hydrogen gas extracted for use in a hydrogen fuel cell. Gas storage in activated carbons is an appealing gas storage method because the gas can be stored in a low pressure, low mass, low volume environment that would be much more feasible than bulky on board compression tanks in vehicles. The United States Department of Energy has specified certain goals to be achieved in the area of research and development of nano-porous carbon materials. As of yet all of the goals are yet to be satisfied but numerous institutions, including the Alliance for Collaborative Research in Alternative Fuel Technology (ALL-CRAFT, http://all-craft.missouri.edu) program, are continuing to conduct work in this promising field.
Filters with activated carbon are usually used in compressed air and gas purification to remove oil vapors, odors, and other hydrocarbons from the air. The most common designs use a 1 stage or 2 stage filtration principle in which activated carbon is embedded inside the filter media. Activated charcoal is also used in spacesuit Primary Life Support Systems. Activated charcoal filters are used to retain radioactive gases from a nuclear boiling water reactor turbine condenser. The air vacuumed from the condenser contains traces of radioactive gases. The large charcoal beds adsorb these gases and retains them while they rapidly decay to non-radioactive solid species. The solids are trapped in the charcoal particles, while the filtered air passes through.
One major industrial application involves use of activated carbon in metal finishing for purification of electroplating solutions. For example, it is the main purification technique for removing organic impurities from bright nickel plating solutions. A variety of organic chemicals are added to plating solutions for improving their deposit qualities and for enhancing properties like brightness, smoothness, ductility, etc. Due to passage of direct current and electrolytic reactions of anodic oxidation and cathodic reduction, organic additives generate unwanted breakdown products in solution. Their excessive build up can adversely affect plating quality and physical properties of deposited metal. Activated carbon treatment removes such impurities and restores plating performance to the desired level.
Activated carbon is used to treat poisonings and overdoses following oral ingestion.
It is thought to bind to poison and prevent its absorption by the gastrointestinal tract. In cases of suspected poisoning, medical personnel administer activated charcoal on the scene or at a hospital's emergency department. Dosing is usually empirical at 1 gram/kg of body weight (for adolescents or adults, give 50–100 g), usually given only once, but depending on the drug taken, it may be given more than once. In rare situations activated charcoal is used in Intensive Care to filter out harmful drugs from the blood stream of poisoned patients. Activated charcoal has become the treatment of choice for many poisonings, and other decontamination methods such as ipecac-induced emesis or stomach pumping are now used rarely.
Activated charcoal for medical use
While activated carbon is useful in acute poisoning, it has been shown to not be effective in long term accumulation of toxins, such as with the use of toxic herbicides.
Mechanisms of action:
•Binding of the toxin to prevent stomach and intestinal absorption. Binding is reversible so a cathartic such as sorbitol may be added as well.
•It interrupts the enterohepatic and enteroenteric circulation of some drugs/toxins and their metabolites
Incorrect application (e.g. into the lungs) results in pulmonary aspiration which can sometimes be fatal if immediate medical treatment is not initiated. The use of activated charcoal is contraindicated when the ingested substance is an acid, an alkali, or a petroleum product.
For pre-hospital (paramedic) use, it comes in plastic tubes or bottles, commonly 12.5 or 25 grams, pre-mixed with water. The trade names include InstaChar, SuperChar, Actidose, Charcodote, and Liqui-Char, but it is commonly called activated charcoal.
Ingestion of activated charcoal prior to consumption of alcoholic beverages appeared to reduce absorption of ethanol into the blood. 5 to 15 milligrams of charcoal per kilogram of body weight taken at the same time as 170 ml of pure ethanol (which equals to about 10 servings of an alcoholic beverage), over the course of one hour, seemed to reduce potential blood alcohol content. Yet other studies showed that this is not the case, and that ethanol blood concentrations were increased because of activated charcoal use.
Charcoal biscuits were sold in England starting in the early 19th century, originally as an antidote to flatulence and stomach trouble.
Tablets or capsules of activated charcoal are used in many countries as an over-the-counter drug to treat diarrhea, indigestion, and flatulence. There is some evidence of its effectiveness as a treatment for irritable bowel syndrome (IBS), and to prevent diarrhea in cancer patients who have received irinotecan. It can interfere with the absorbency of some medications, and lead to unreliable readings in medical tests such as the guaiac card test. Activated charcoal is also used for bowel preparation by reducing intestinal gas content before abdominal radiography to visualize bile and pancreatic and renal stones. A type of charcoal biscuit has also been marketed as a pet care product.
Activated carbon, often impregnated with iodine or sulfur, is widely used to trap mercury emissions from coal-fired power stations, medical incinerators, and from natural gas at the wellhead. This carbon is a specialty product costing more than US$4.00 per kg. However, it is often not recycled.
Disposal in the USA after absorbing mercury
The mercury laden activated carbon presents a disposal dilemma. If the activated carbon contains less than 260 ppm mercury, Federal regulations allow it to be stabilized (for example, trapped in concrete) for land filling However, waste containing greater than 260 ppm is considered to be in the high mercury subcategory and is banned from land filling (Land-Ban Rule). It is this material which is now accumulating in warehouses and in deep abandoned mines at an estimated rate of 1000 tons per year.
The problem of disposal of mercury laden activated carbon is not unique to the U.S. In the Netherlands this mercury is largely recovered and the activated carbon is disposed by complete burning.
- Heterogeneous Catalysis
The most commonly encountered form of chemisorption in industry occurs when a solid catalyst interacts with a gaseous feedstock, the reactant/s. The adsorption of reactant/s to the catalyst surface creates a chemical bond, altering the electron density around the reactant molecule and allowing it to undergo reactions that would not normally be available to it. - Adsorption Refrigeration
Adsorption refrigeration and heat pump cycles rely on the adsorption of a refrigerant gas into an adsorbent at low pressure and subsequent desorption by heating. The adsorbent acts as a "chemical compressor" driven by heat and is, from this point of view, the "pump" of the system. It consists of a solar collector, a condenser or heat-exchanger and an evaporator that is placed in a refrigerator box. The inside of the collector is lined with an adsorption bed packed with activated carbon adsorbed with methanol. The refrigerator box is insulated filled with water. The activated carbon can adsorb a large amount of methanol vapors in ambient temperature and desorb it at a higher temperature (around 100 degrees Celsius). During the daytime, the sunshine irradiates the collector, so the collector is heated up and the methanol is desorbed from the activated carbon. In desorption, the liquid methanol adsorbed in the charcoal heats up and vaporizes. The methanol vapour condenses and is stored in the evaporator.At night, the collector temperature decreases to the ambient temperature, and the charcoal adsorbs the methanol from the evaporator. The liquid methanol in the evaporator vaporizes and absorbs the heat from the water contained in the trays. Since adsorption is a process of releasing heat, the collector must be cooled efficiently at night. As mentioned above, the adsorption refrigeration system operates in an intermittent way to produce the refrigerating effect. Helium gas can also be 'pumped' by thermally cycling activated carbon 'sorption pumps' between 4 kelvins and higher temperatures. An example of this is to provide the cooling power for the Oxford Instruments AST series dilution refrigerators. 3He vapor is pumped from the surface of the dilute phase of a mixture of liquid 4He and its isotope 3He. The 3He is adsorbed onto the surfaces of the carbon at low temperature (typically <4K), the regeneration of the pump between 20 and 40 K returns the 3He to the concentrated phase of the liquid mixture. Cooling occurs at the interface between the two liquid phases as 3He 'evaporates' across the phase boundary. If more than one pump is present in the system a continuous flow of gas and hence constant cooling power can be obtained, by having one sorption pump regenerating while the other is pumping. Systems such as this allow temperatures as low as 10 mK (0.01 kelvin) to be obtained with very few moving parts.
The reactivation or the regeneration of activated carbons involves restoring the adsorptive capacity of saturated activated carbon by desorbing adsorbed contaminants on the activated carbon surface.
Thermal regeneration or reactivation
The most common regeneration technique employed in industrial processes is thermal regeneration. The thermal regeneration process generally follows three steps:
• Adsorbent drying at approximately 105 °C (221 °F)
• High temperature desorption and decomposition 500–900 °C (932–1,652 °F) under an inert atmosphere
• Residual organic gasification by an oxidizing gas (steam or carbon dioxide) at elevated temperatures 800 °C (1,470 °F)
The heat treatment stage utilizes the exothermic nature of adsorption and results in desorption, partial cracking and polymerization of the adsorbed organics. The final step aims to remove charred organic residue formed in the porous structure in the previous stage and re-expose the porous carbon structure regenerating its original surface characteristics. After treatment the adsorption column can be reused. Per adsorption-thermal regeneration cycle between 5–15 wt% of the carbon bed is burnt off resulting in a loss of adsorptive capacity. Thermal regeneration is a high energy process due to the high required temperatures making it both an energetically and commercially expensive process. Plants that rely on thermal regeneration of activated carbon have to be of a certain size before it is economically viable to have regeneration facilities onsite. As a result it is common for smaller waste treatment sites to ship their activated carbon cores to a specialized facility for regeneration.
Other regeneration techniques
Current concerns with the high energy/cost nature of thermal regeneration of activated carbon has encouraged research into alternative regeneration methods to reduce the environmental impact of such processes. Though several of the regeneration techniques cited have remained areas of purely academic research, some alternatives to thermal regeneration systems have been employed in industry. Current alternative regeneration methods are:
• Chemical and solvent regeneration
• Microbial regeneration
• Electrochemical regeneration
• Ultrasonic regeneration
• Wet air oxidation
Others have attempted to extract the highest value from scrap tire recycling with pyrolysis. Profitable Pyrolysis faces Two challenges: design, costs and operations of tire pyrolysis plants at industrial scale; and the commodity nature of the by-products. We have a solution that have overcome all these obstacles. And we are in production.
- Chada, Nagaraju; Romanos, Jimmy; Hilton, Ramsey; Suppes, Galen; Burress, Jacob; Pfeifer, Peter (2012-03-01). "Activated carbon monoliths for methane storage". Bulletin of the American Physical Society. 57 (1): W33.012. Bibcode:2012APS..MARW33012C.
- Soo, Yuchoong; Chada, Nagaraju; Beckner, Matthew; Romanos, Jimmy; Burress, Jacob; Pfeifer, Peter (2013-03-20). "Adsorbed Methane Film Properties in Nanoporous Carbon Monoliths". Bulletin of the American Physical Society. 58 (1): M38.001. Bibcode:2013APS..MARM38001S.
- ""Properties of Activated Carbon", CPL Caron Link, accessed 2008-05-02". Archived from the original on 19 June 2012. Retrieved 13 October 2014.
- Dillon, Edward C; Wilton, John H; Barlow, Jared C; Watson, William A (1989-05-01). "Large surface area activated charcoal and the inhibition of aspirin absorption". Annals of Emergency Medicine. 18 (5): 547–552. doi:10.1016/S0196-0644(89)80841-8. PMID 2719366.
- P. J. Paul. "Value Added Products from Gasification – Activated Carbon" (PDF). Bangalore: The Combustion, Gasification and Propulsion Laboratory (CGPL) at the Indian Institute of Science (IISc).
- IBM Micromedex (1 February 2019). "Charcoal, Activated (Oral Route)". Mayo Clinic. Retrieved 15 February 2019.
- World Health Organization (2019). World Health Organization model list of essential medicines: 21st list 2019. Geneva: World Health Organization. hdl:10665/325771. WHO/MVP/EMP/IAU/2019.06. License: CC BY-NC-SA 3.0 IGO.
- "Charcoal, Activated". The American Society of Health-System Pharmacists. Retrieved 23 April 2014.
- Elliott CG, Colby TV, Kelly TM, Hicks HG (1989). "Charcoal lung. Bronchiolitis obliterans after aspiration of activated charcoal". Chest. 96 (3): 672–4. doi:10.1378/chest.96.3.672. PMID 2766830.
- Exner, T; Michalopoulos, N; Pearce, J; Xavier, R; Ahuja, M (March 2018). "Simple method for removing DOACs from plasma samples". Thrombosis Research. 163: 117–122. doi:10.1016/j.thromres.2018.01.047. PMID 29407622.
- Exner, T; Ahuja, M; Ellwood, L (24 April 2019). "Effect of an activated charcoal product (DOAC Stop™) intended for extracting DOACs on various other APTT-prolonging anticoagulants". Clinical Chemistry and Laboratory Medicine. 57 (5): 690–696. doi:10.1515/cclm-2018-0967. PMID 30427777.
- "Activated Carbon | Solvent Recovery | VOC Abatement Systems". DEC IMPIANTI. Retrieved 2019-10-20.
- EPA Alumni Association: Senior EPA officials discuss early implementation of the Safe Drinking Water Act of 1974, Video, Transcript (see pages 15-16).
- "Activated Carbon | Solvent Recovery | Abatement Systems". DEC IMPIANTI. Retrieved 2019-10-21.
- Activated Charcoal Review Sheet, USDA Organic Materials Review, February 2002.
- Activated Carbon Petition, USDA Organic Materials Review petition, Canadaigua Wine, May 2002.
- "Alliance for Collaborative Research in Alternative Fuel Technology". All-craft.missouri.edu. Retrieved 2014-03-13.
- Bourke, Marta (1989). "Activated Carbon for Mercury Removal". Archived from the original on 2013-08-03. Retrieved 2013-08-27.
- Tim Flannery, Here On Earth: A New Beginning, Allen Lane (2011), p. 186.
- J.Romanos; et al. (2012). "Nanospace engineering of KOH activated carbon". Nanotechnology. 23 (1): 015401. Bibcode:2012Nanot..23a5401R. doi:10.1088/0957-4484/23/1/015401. PMID 22156024.
- The separation of the most volatile gases from air without liquefaction
- "SentryAir". SentryAir. Retrieved 2014-03-13.
- TIGG Corporation. Granular activated carbon selection Archived 2012-09-12 at the Wayback Machine. Published 2012-05-8, retrieved 2012-09-21.
- Philippe Serp, José Luis Figueiredo, Carbon Materials for Catalysis, Wiley, – 2009, – 550 p.
- Gómez-Serrano, V.; Piriz-Almeida, F. N.; Durán-Valle, C. J.; Pastor-Villegas, J. (1999). "Formation of oxygen structures by air activation. A study by FT-IR spectroscopy". Carbon. 37 (10): 1517–1528. doi:10.1016/S0008-6223(99)00025-1.
- Machnikowski J.; Kaczmarska H.; Gerus-Piasecka I.; Diez M.A.; Alvarez R.; Garcia R. (2002). "Structural modification of coal-tar pitch fractions during mild oxidation – relevance to carbonization behavior". Carbon. 40 (11): 1937–1947. doi:10.1016/s0008-6223(02)00029-5.
- Petrov N.; Budinova T.; Razvigorova M.; Ekinci E.; Yardim F.; Minkova V. (2000). "Preparation and characterization of carbon adsorbents from furfural". Carbon. 38 (15): 2069–2075. doi:10.1016/s0008-6223(00)00063-4.
- arcia A.B.; Martinez-Alonso A.; Leon C. A.; Tascon J.M.D. (1998). "Modification of the surface properties of an activated carbon by oxygen plasma treatment". Fuel. 77 (1): 613–624. doi:10.1016/S0016-2361(97)00111-7.
- Saha B.; Tai M.H.; Streat M. (2001). "Study of activated carbon after oxidation and subsequent treatment characterization". Process Safety and Environmental Protection. 79 (4): 211–217. doi:10.1205/095758201750362253.
- Polovina M.; Babic B.; Kaluderovic B.; Dekanski A. (1997). "Surface characterization of oxidized activated carbon cloth". Carbon. 35 (8): 1047–1052. doi:10.1016/s0008-6223(97)00057-2.
- Fanning P.E.; Vannice M.A. (1993). "A DRIFTS study of the formation of surface groups on carbon by oxidation". Carbon. 31 (5): 721–730. doi:10.1016/0008-6223(93)90009-y.
- Youssef A.M.; Abdelbary E.M.; Samra S.E.; Dowidar A.M. (1991). "Surface-properties of carbons obtained from polyvinyl-chloride". Ind. J. Chem. A. 30 (10): 839–843.
- Arriagada R.; Garcia R.; Molina-Sabio M.; Rodriguez-Reinoso F. (1997). "Effect of steam activation on the porosity and chemical nature of activated carbons from Eucalyptus globulus and peach stones". Microporous Mat. 8 (3–4): 123–130. doi:10.1016/s0927-6513(96)00078-8.
- Molina-Sabio M.; Gonzalez M.T.; Rodriguez-Reinoso F.; Sepulveda-Escribano A. (1996). "Effect of steam and carbon dioxide activation in the micropore size distribution of activated carbon". Carbon. 34 (4): 505–509. doi:10.1016/0008-6223(96)00006-1.
- Bradley RH, Sutherland I, Sheng E (1996). "Carbon surface: Area, porosity, chemistry, and energy". Journal of Colloid and Interface Science. 179 (2): 561–569. Bibcode:1996JCIS..179..561B. doi:10.1006/jcis.1996.0250.
- Sutherland I.; Sheng E.; Braley R.H.; Freakley P.K. (1996). "Effects of ozone oxidation on carbon black surfaces". J. Mater. Sci. 31 (21): 5651–5655. Bibcode:1996JMatS..31.5651S. doi:10.1007/bf01160810.
- Rivera-Utrilla J, Sanchez-Polo M (2002). "The role of dispersive and electrostatic interactions in the aqueous phase adsorption of naphthalenesulphonic acids on ozone-treated activated carbons". Carbon. 40 (14): 2685–2691. doi:10.1016/s0008-6223(02)00182-3.
- Valdés, H.; Sánchez-Polo, M.; Rivera-Utrilla, J.; Zaror, C. A. (2002). "Effect of Ozone Treatment on Surface Properties of Activated Carbon". Langmuir. 18 (6): 2111–2116. doi:10.1021/la010920a.
- Pradhan B.K.; Sandle N.K. (1999). "Effect of different oxidizing agent treatments on the surface properties of activated carbons". Carbon. 37 (8): 1323–1332. doi:10.1016/s0008-6223(98)00328-5.
- Acedo-Ramos M.; Gomez-Serrano V.; Valenzuella-Calahorro C.; Lopez-Peinado A.J. (1993). "Oxydation of activated carbon in liquid phase. Study by FT-IR". Spectroscopy Letters. 26 (6): 1117–1137. Bibcode:1993SpecL..26.1117A. doi:10.1080/00387019308011598.
- Gomez-Serrano V.; Acedo-Ramos M.; Lopez-Peinado A.J.; Valenzuela-Calahorro C. (1991). "Stability towards heating and outgassing of activated carbon oxidized in the liquid-phase". Thermochimica Acta. 176: 129–140. doi:10.1016/0040-6031(91)80268-n.
- Stőhr B.; Boehm H.P.; Schlőgl R. (1991). "Enhancement of the catalytic activity of activated carbons in oxidation reactions by termal treatment with ammonia or hydrogen cyanide and observation of a superoxide species as a possible intermediate". Carbon. 29 (6): 707–720. doi:10.1016/0008-6223(91)90006-5.
- Biniak S.; Szymański G.; Siedlewski J.; Światkowski A. (1997). "The characterizaíion of activated carbons with oxygen and nitrogen surface groups". Carbon. 35 (12): 1799–1810. doi:10.1016/s0008-6223(97)00096-1.
- Boudou J.P.; Chehimi M.; Broniek E.; Siemieniewska T.; Bimer J. (2003). "Adsorption of H2S or SO2 on an activated carbon cloth modified by ammonia treatment" (PDF). Carbon. 41 (10): 1999–2007. doi:10.1016/s0008-6223(03)00210-0.
- Sano H.; Ogawa H. (1975). "Preparation and application nitrogen containing active carbons". Osaka Kogyo Gijutsu Shirenjo. 26 (5): 2084–2086.
- Radkevich, V. Z.; Senko, T. L.; Wilson, K.; Grishenko, L. M.; Zaderko, A. N.; Diyuk, V. Y. (2008). "The influence of surface functionalization of activated carbon on palladium dispersion and catalytic activity in hydrogen oxidation". Applied Catalysis A: General. 335 (2): 241–251. doi:10.1016/j.apcata.2007.11.029.
- Evans, M. J. B.; Halliop, E.; Liang, S.; MacDonald, J. A. F. (1998). "The effect of chlorination on surface properties of activated carbon". Carbon. 36 (11): 1677–1682. doi:10.1016/S0008-6223(98)00165-1.
- Papirer, E. N.; Lacroix, R.; Donnet, J. B.; Nansé, G. R.; Fioux, P. (1995). "XPS study of the halogenation of carbon black—Part 2. Chlorination". Carbon. 33: 63–72. doi:10.1016/0008-6223(94)00111-C.
- Papirer, Eugène; Lacroix, Renaud; Donnet, Jean-Baptiste; Nanse, Gérard; Fioux, Philippe (1994). "XPS Study of the halogenation of carbon black-part 1. Bromination". Carbon. 32 (7): 1341–1358. doi:10.1016/0008-6223(94)90121-X.
- Nansé, G.; Papirer, E.; Fioux, P.; Moguet, F.; Tressaud, A. (1997). "Fluorination of carbon blacks: An X-ray photoelectron spectroscopy study: III. Fluorination of different carbon blacks with gaseous fluorine at temperatures below 100 °C influence of the morphology, structure and physico-chemical characteristics of the carbon black on the fluorine fixation". Carbon. 35 (4): 515–528. doi:10.1016/S0008-6223(97)00003-1.
- US8648217B2, "Modification of carbonaceous materials", issued 2008-08-04
- US10000382B2, "Method for carbon materials surface modification by the fluorocarbons and derivatives", issued 2015-11-03
- aderko, Alexander N.; Shvets, Roman Ya.; Grygorchak, Ivan I.; Afonin, Sergii; Diyuk, Vitaliy E.; Mariychuk, Ruslan T.; Boldyrieva, Olga Yu.; Kaňuchová, Mária; Lisnyak, Vladyslav V. (2018-11-20). "Fluoroalkylated Nanoporous Carbons: Testing as a Supercapacitor Electrode". Applied Surface Science. 470: 882–892. doi:10.1016/j.apsusc.2018.11.141. ISSN 0169-4332.
- Aldana-Pérez, A.; Lartundo-Rojas, L.; Gómez, R.; Niño-Gómez, M. E. (2012). "Sulfonic groups anchored on mesoporous carbon Starbons-300 and its use for the esterification of oleic acid". Fuel. 100: 128–138. doi:10.1016/j.fuel.2012.02.025.
- Diyuk, V. E.; Zaderko, A. N.; Grishchenko, L. M.; Yatsymyrskiy, A. V.; Lisnyak, V. V. (2012). "Efficient carbon-based acid catalysts for the propan-2-ol dehydration". Catalysis Communications. 27: 33–37. doi:10.1016/j.catcom.2012.06.018.
- "WO18194533 METHOD FOR CHEMICAL MODIFICATION OF FLUORINATED CARBONS WITH SULFUR-CONTAINING SUBSTANCE". patentscope.wipo.int. Retrieved 2018-11-24.
- Budarin, V. L.; Clark, J. H.; Tavener, S. J.; Wilson, K. (2004). "Chemical reactions of double bonds in activated carbon: Microwave and bromination methods". Chemical Communications (23): 2736–7. doi:10.1039/B411222A. PMID 15568092.
- Bagreev, A.; Rhaman, H.; Bandosz, T. J (2001). "Thermal regeneration of a spent activated carbon adsorbent previously used as hydrogen sulfide adsorbent". Carbon. 39 (9): 1319–1326. doi:10.1016/S0008-6223(00)00266-9.
- Sabio, E.; Gonzalez, E.; Gonzalez, J. F.; Gonzalez-Garcia, C. M.; Ramiro, A.; Ganan, J (2004). "Thermal regeneration of activated carbon saturated with p-nitrophenol". Carbon. 42 (11): 2285–2293. doi:10.1016/j.carbon.2004.05.007.
- Miguel GS, Lambert SD, Graham NJ (2001). "The regeneration of field spent granular activated carbons". Water Research. 35 (11): 2740–2748. doi:10.1016/S0043-1354(00)00549-2. PMID 11456174.
- Alvarez PM, Beltrán FJ, Gómez-Serrano V, Jaramillo J, Rodríguez EM (2004). "Comparison between thermal and ozone regenerations of spent activated carbon exhausted with phenol". Water Research. 38 (8): 2155–2165. doi:10.1016/j.watres.2004.01.030. PMID 15087197.
- Martin, R. J.; Wj, N (1997). "The repeated exhaustion and chemical regeneration of activated carbon". Water Research. 21 (8): 961–965. doi:10.1016/S0043-1354(87)80014-3.
- Aizpuru A, Malhautier L, Roux JC, Fanlo JL (2003). "Biofiltration of a mixture of volatile organic compounds on granular activated carbon". Biotechnology and Bioengineering. 83 (4): 479–488. doi:10.1002/bit.10691. PMID 12800142.
- Narbaitz RM, Karimi-Jashni A (2009). "Electrochemical regeneration of granular activated carbons loaded with phenol and natural organic matter". Environmental Technology. 30 (1): 27–36. doi:10.1080/09593330802422803. PMID 19213463.
- Lim JL, Okada M (2005). "Regeneration of granular activated carbon using ultrasound". Ultrasonic-Sono-Chemistry. 12 (4): 277–285. doi:10.1016/j.ultsonch.2004.02.003. PMID 15501710.
- Shende RV, Mahajani VV (2002). "Wet oxidative regeneration of activated carbon loaded with reactive dye". Waste Management. 22 (1): 73–83. doi:10.1016/S0956-053X(01)00022-8. PMID 11942707.
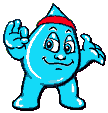
We Want To Hear From You
Let us know if you have any questions about our products and services or if you would like to get a quote!
Phone and Email
Phone: +1 (719) 4759919
Email: spencerpugh@earthlink.net